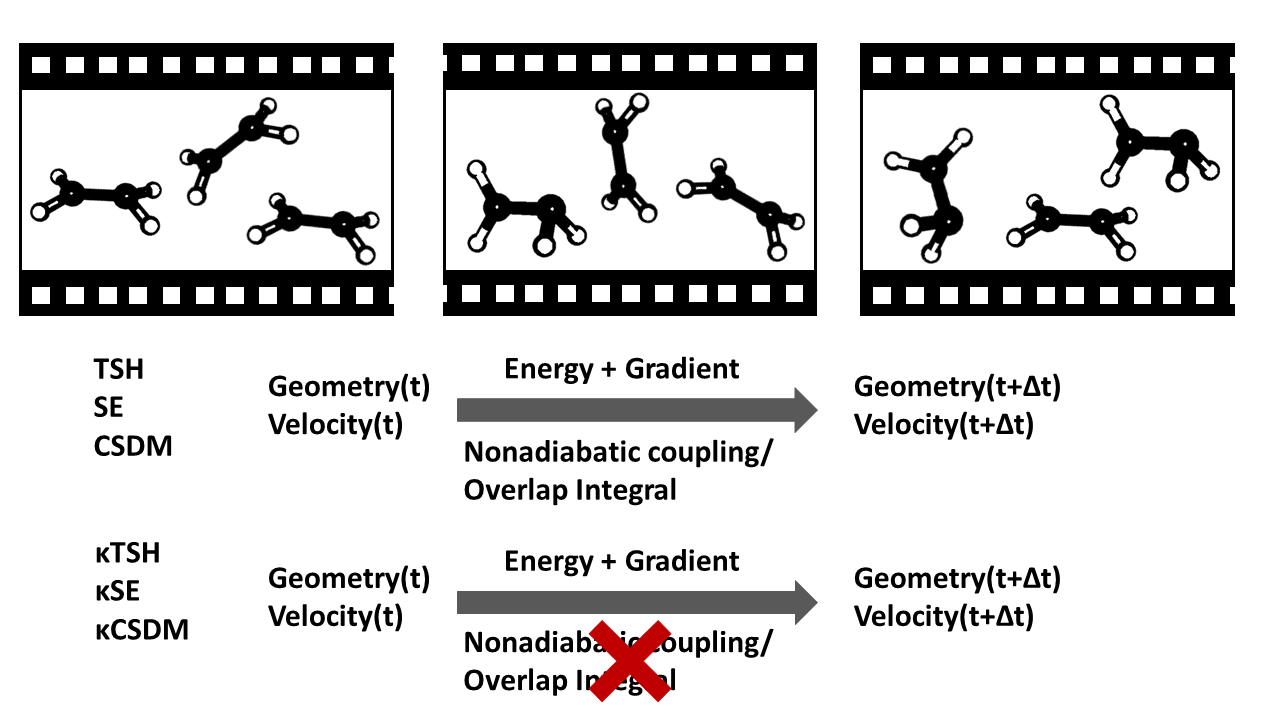
Truhlar Research Group News
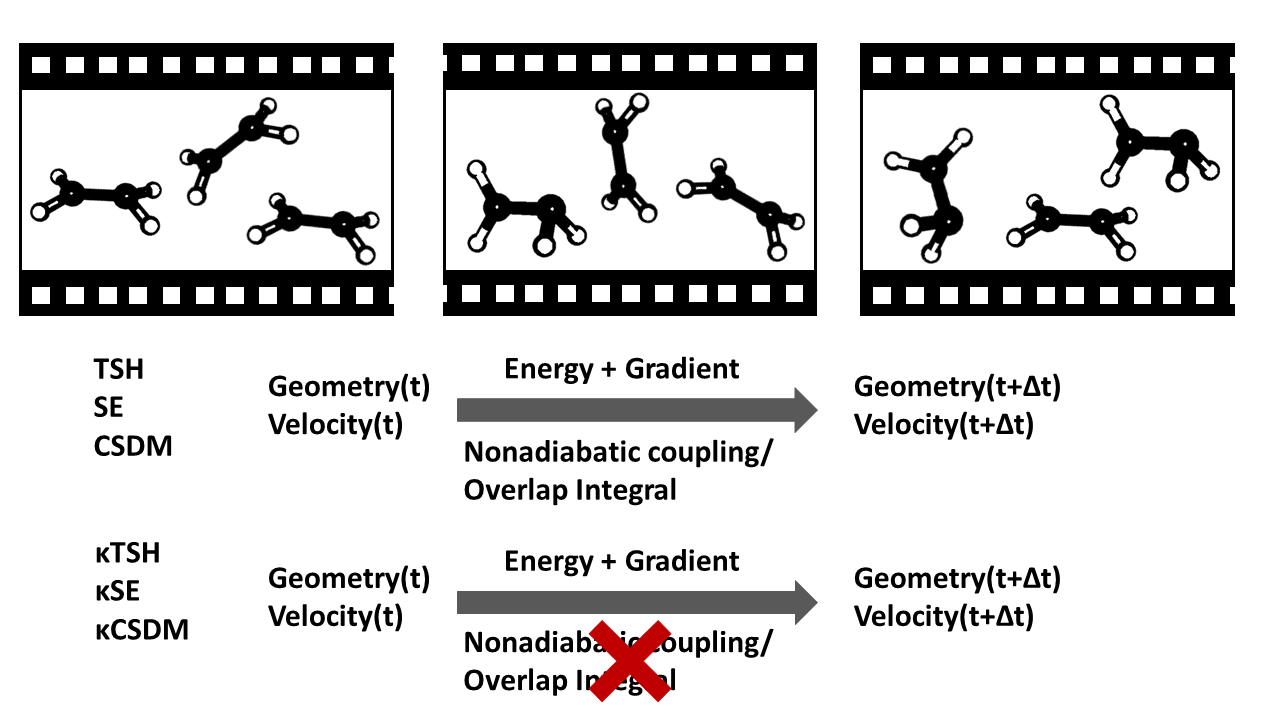
Whenever possible one prefers to perform dynamical calculations by direct dynamics, in which potential energies, their derivatives, and potential surface couplings are computed on demand by electronic structure calculations carried out side-by-side with the dynamics algorithm. For electronically nonadiabatic processes, this requires not only energies but also nonadiabatic coupling vectors (NACs), which are defined as
$$\mathbf{d}_{I J}=\left\langle\phi_I\middle|\partial / \partial \mathbf{R}\middle| \phi_J\right\rangle$$
where \(\left\langle\ldots\middle|\ldots\middle|\ldots\right\rangle\) denotes an electronic matrix element, \(\phi_I\) and \(\phi_J\) are electronic eigenfunctions, and the operator is a \(3N_\textrm{atoms}\)-dimensional gradient with respect to nuclear coordinates. The evaluation of the NACs adds to the cost, and it is often inconvenient. For example, some software will produce the electronic energies but not the NACs, and for some methods of predicting electronic energies, the NACs are unavailable because the method predicts energies without an explicit wave function corresponding to the energies. In addition to expense and inconvenience, even when NACs are available, they present problems due to origin dependence, spurious long-range couplings, and artificial translational and rotational components.
In some semiclassical multi-surface trajectory methods, like trajectory surface hopping (TSH), one only needs the component of the NAC along the current velocity direction, and in such cases one can use the time derivative of the electronic wave function along the trajectory instead of the NAC. Although, when possible, this is more efficient, it would be even more convenient to have approximations to NACs that can be evaluated straightforwardly from energies and energetic derivatives without gradients of electronic wave functions or time derivatives of electronic wave functions. In other more accurate dynamics methods, like quantum dynamics or coherent switching with decay of mixing (CSDM), one needs the whole NAC. We therefore asked, can we carry out electronically nonadiabatic dynamics using only energy information, and does this provide enough accuracy for efficient propagation of multi-surface molecular dynamics? We were able to answer this question, “YES – NACs for free!”. This work is now published:
“Nonadiabatic Dynamics Algorithms with Only Potential Energies and Gradients: Curvature-Driven Coherent Switching with Decay of Mixing and Curvature-Driven Trajectory Surface Hopping,” Y. Shu, L. Zhang, X. Chen, S. Sun, Y. Huang, and D. G. Truhlar, Journal of Chemical Theory and Computation 18, 1320-1328 (2022). doi.org/10.1021/acs.jctc.1c01080
In the above work, starting with the curvature-based approximation of Baeck and An for one-dimensional systems, we proposed a curvature-approximated time-derivative coupling formula and effective NAC applicable to polyatomic dynamics. (In independent work carried out at about the same time, do Casal et al. [doi.org/10.12688/openreseurope.13624.1] also extended the Baeck–An approximation to multiple dimensions, but in their case only for the component of the NAC along the velocity vector.) We showed how to use the time-derivative coupling formula and effective NACs to obtain curvature-driven versions of CSDM and TSH with energy-based decoherence (TSH-EDC). These curvature-driven methods are respectively called κCSDM and κTSH-EDC. In the original paper (reference above) we compared S1 population decays calculated by κCSDM to those calculated by CSDM based on NACs and CSDM based on time-derivative coupling (approximated by overlap integrals of electronic wave functions). These calculations employed SA-CASSCF electronic wave functions, and the half-lives of the three dynamical methods agree within the statistical uncertainty due to Monte Carlo sampling over initial conditions of the ensemble.
A similar comparison of SA-CASSCF S1 population decays was made for ammonia:
“Direct Nonadiabatic Dynamics of Ammonia with Curvature-Driven Coherent Switching with Decay of Mixing and with Fewest Switches with Time Uncertainty: An Illustration of Population Leaking in Trajectory Surface Hopping Due to Frustrated Hops,” X. Zhao, Y. Shu, L. Zhang, X. Xu, and D. G. Truhlar, Journal of Chemical Theory and Computation 23, 1672-1685 (2023). doi.org/10.1021/acs.jctc.2c01260
Good agreement was found between κCSDM and CSDM based on NACs.
Another check of κCSDM was carried out for photodissociation of 1,3-cyclohexadiene:
“Nonadiabatic Dynamics of 1,3-Cyclohexadiene by Curvature-Driven Coherent Switching with Decay of Mixing,” L. Zhang, Y. Shu, S. Bhaumik, X. Chen, S. Sun, Y. Huang, and D. G. Truhlar, Journal of Chemical Theory and Computation 18, 7073-7081 (2022). doi.org/10.1021/acs.jctc.2c00801
In this work we compared 42-dimensional 3-state κCSDM calculations based on XMS-CASPT2 wave functions to experiment and to earlier dynamics calculations employing NACs [Polyak et al., doi.org/10.1021/acs.jctc.9b00396], and again good agreement was obtained.
Systems with dense manifolds of electronically excited states can be challenging for electronically nonadiabatic dynamics. We compared κCSDM calculations to CSDM calculations based on NACs for total electronically nonadiabatic cross sections in high-energy 5A´collisions of ground-electronic state O2 with ground state O:
Semiclassical Multi-State Dynamics for Six Coupled 5A′ States of O + O2,” F. B. Akher, Y. Shu, Z. Varga, and D. G. Truhlar, Journal of Chemical Theory and Computation 19, 4389-4401 (2023). doi.org/10.1021/acs.jctc.3c00517
These calculations, unlike the ones already discussed, were not direct dynamics but rather were based on analytic fits to diabatic potential energy matrices based on input from 6-state XMS-CASPT2 calculations. We found very good agreement for 30 sets of initial translational and vibrational energies.
Concluding remarks
The curvature-driven methods do not require the calculations of NACs or time derivatives of the wave function. There are no spurious long-range couplings, no origin dependence, no artificial breaking of the conservation of center of mass motion and nuclear angular momentum, no phase issues, and no added expense. Electronically nonadiabatic dynamics calculations agree well with calculations based on NACs. The curvature-driven methods can be used for direct dynamics calculations of electronically nonadiabatic processes with electronic structure methods for which NACs or time derivatives are not available. They simply require adiabatic potential energy surfaces and their gradients. Both κCSDM and κTSH-EDC are available in the software packages SHARC 3.0. and SHARC-MN 2.0:
SHARC 3.0: M. Oppel, maisebastian, and schnarc. (2023). sharc-md/sharc: SHARC Release 3.0.1 (v3.0.1). Zenodo. https://doi.org/10.5281/zenodo.7896064
SHARC-MN 2.0: Y. Shu, L. Zhang, and D. G. Truhlar. (2023). SHARC-MN v2.0 (2.0). Zenodo. https://doi.org/10.5281/zenodo.7818894
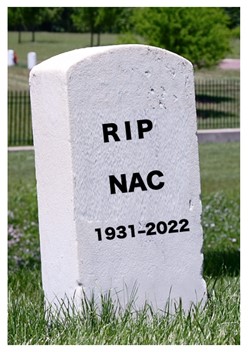
The editors of Chemical Physics Reviews evaluated the article “Computational Quantum Chemistry of Metal–Organic Frameworks” by Indrani Choudhuri, Jingyun Ye, and Donald G. Truhlar [Chem. Phys. Rev. 4, article no. 031304 (2023); doi.org/10.1063/5.0153656] as one of the journal’s best, and they chose to promote it as a Feature Article. The article is discussed in “Computational methodologies hunt for elusive metal-organic frameworks” by Amy Thompson [Scilight 2023 (32); doi.org/10.1063/10.0020716].
Metal–organic frameworks (MOFs) exhibit exceptional properties suitable for a wide range of applications, including gas separation and storage, heterogeneous catalysis, photocatalysis, and electrocatalysis. Computational modeling has emerged as a valuable and efficient tool for comprehending and designing MOFs and explicating their functional behavior. In this review, we outline various computational methodologies, especially Kohn–Sham density functional theory, for determining the structural, electronic, and magnetic properties of MOFs, and we survey selected illustrative applications of these methods. The combination of state-of-the-art computational methods reviewed in the article with more comprehensive experimental data obtained through advanced instrumentation and characterization techniques is expected to yield a deeper understanding of current MOF applications and enable rational design of MOFs with enhanced functionalities.
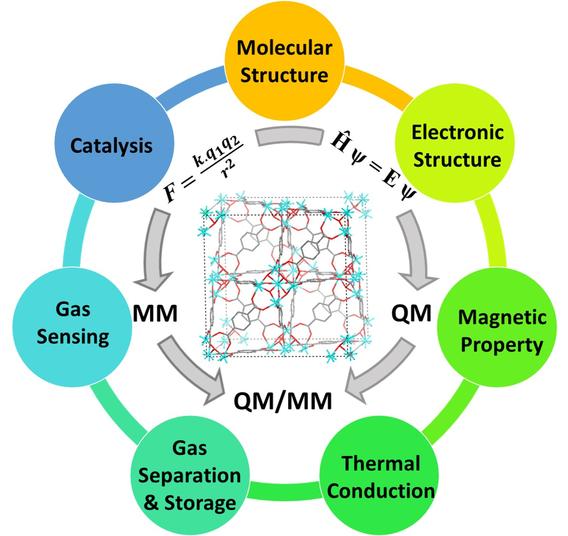
Several former group members and friends of the group attended the 33rd Chinese Chemical Society Congress in Qingdao this month. The attached photo shows (left to right):
Ke Yang, Naikan University
Xin Zhang, Beijing University of Chemical Technology
Xuefei Xu, Tsinghua University
Peifeng Su, Xiamen University
Xiao He, East China Normal University
Bo Long, Guizhou Minzu University
Wei Lin, Fuzhou University
Xin-Ping Wu, Shanghai Jiao Tong University
Jinfeng Liu, China Pharmaceutical University (previous PhD student of Xiao He)
Our group is very proud of the continued success of all our group alumni and friends.
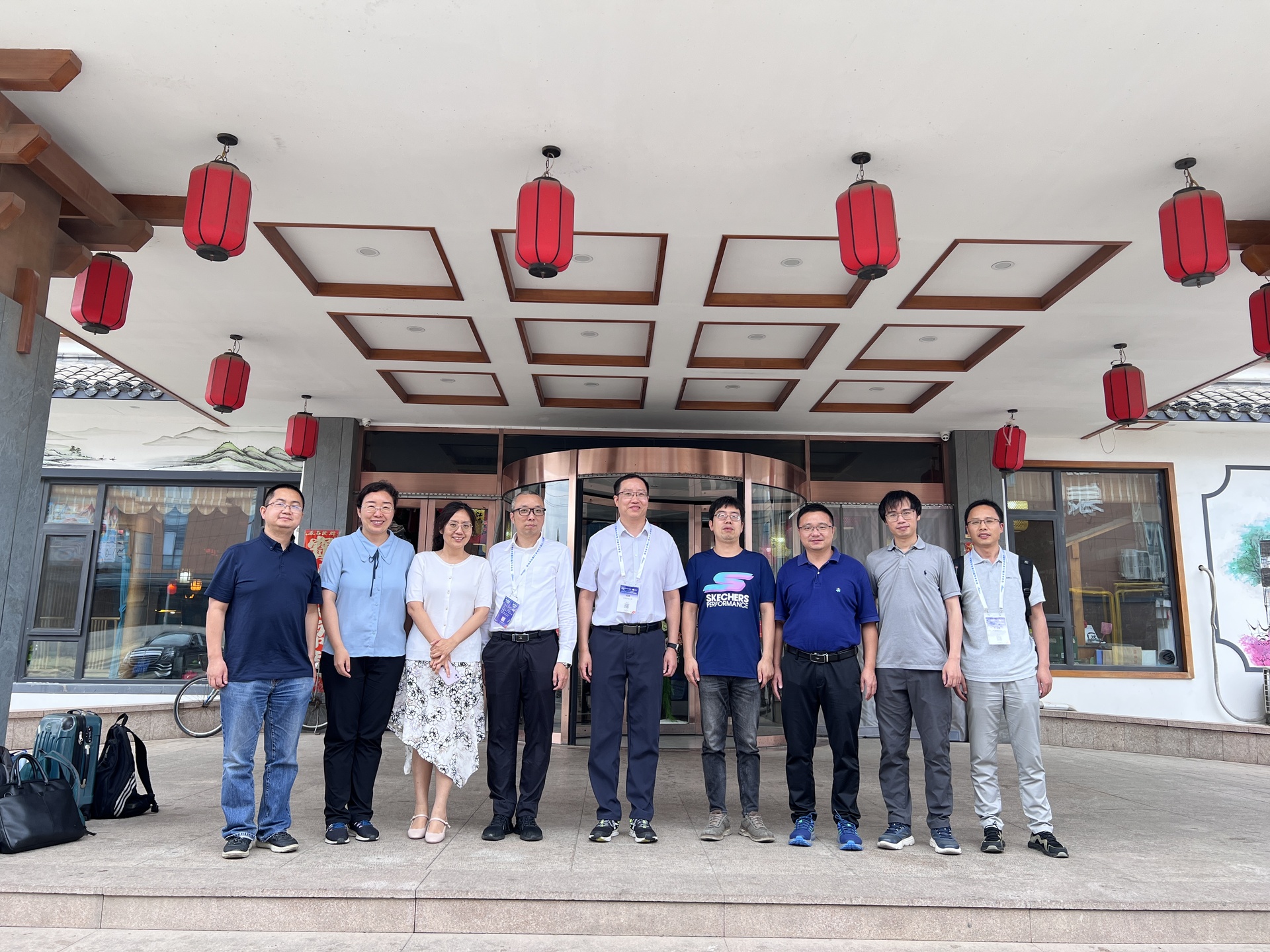
Yesterday, Research.com informed Professor Truhlar that he has been recognized with their Chemistry Leader Award for 2023. Research.com recently released the 2023 Edition of the Ranking of Top Scientists in the field of Chemistry:
https://research.com/scientists-rankings/chemistry
Professor Truhlar ranked 7th in the world and 4th in United States.
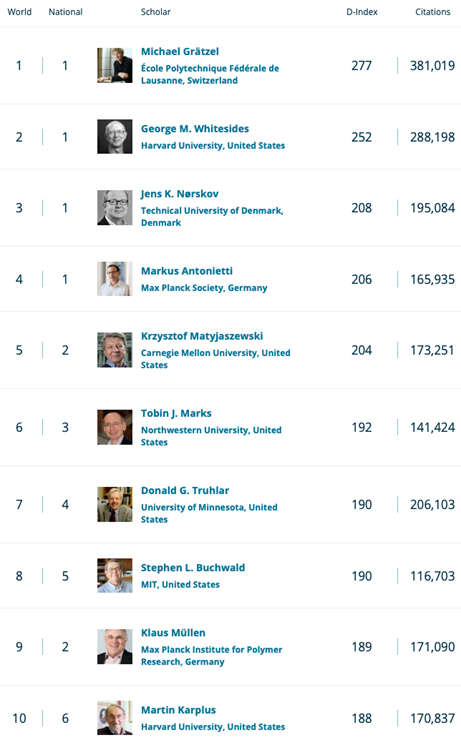
Today was published our invited perspective article in JCTC:
Y. Shu and D. G. Truhlar, Journal of Chemical Theory and Computation 19, 380-395 (2022).
It is openly available to all readers at doi.org/10.1021/acs.jctc.2c00988.
In this perspective, we introduced the theoretical concepts that are essential to understand decoherence in electronically nonadiabatic molecular events. We emphasized that the reduced density matrix of a subsystem evolves according to a nonunitary Liouville–von Neumann equation even if the full density matrix of the combined subsystem and environment remains pure. When governed by a nonunitary Liouville–von Neumann equation, the off-diagonal elements of the subsystem reduced density matrix decay to zero. This is the central fact of decoherence; and this decay of coherence is essential in understanding the propagation of the electronic reduced density matrix in chemical and physical systems, not just in a condensed phase due to the solvent but even in a small molecule in the gas phase, where the electronic subsystem is decohered by the nuclei, which act as an environment. We discussed how the continuous monitoring of electrons by the nuclei causes decoherence of the reduced electronic density matrix.
Simulations of the decoherence effect in nonadiabatic dynamics can be achieved by a combination of a decay-of-mixing algorithm and an ensemble average over initial conditions, for example by decoherence with decay of mixing, which is available in the ANT program and will soon become available in SHARC-3.0.
Decoherence was also recently discussed (very briefly) in Don Truhlar’s letter to the editor of Physics Today: “More on the Quantum Measurement Problem,” D. G. Truhlar, Physics Today 75(11), 13 (Nov. 2022) doi.org/10.1063/PT.3.5113
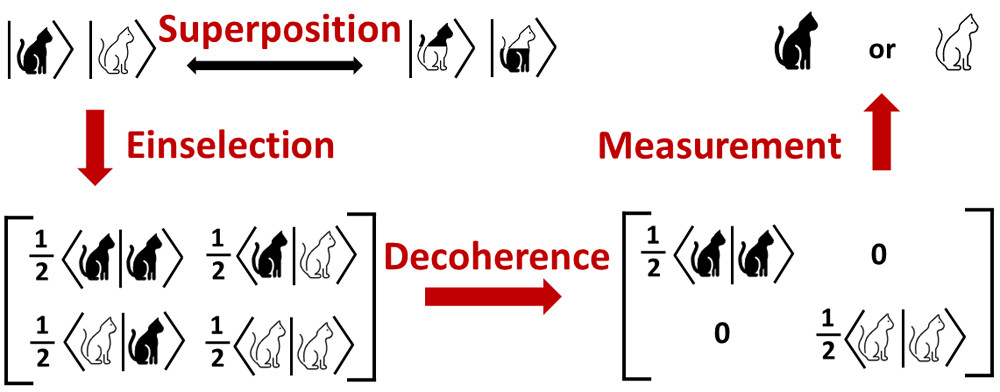
Y. Liu, C. Zhang, Z. Liu, D. G. Truhlar, Y. Wang, and X. He, Nature Computational Science 3, 48-58 (2023).
The paper is available as an open access publication at
doi.org/10.1038/s43588-022-00371-5
Kohn–Sham density functional theory (KS-DFT) has been widely used in various fields of chemistry, but no functional can accurately predict the whole range of chemical properties. A universal functional that has high across-the-board accuracy for both main-group elements and transition metals is highly desirable for a broad range of chemical applications.
In this work, we optimized an exchange-correlation functional, CF22D, with high across-the-board accuracy for chemical applications by using a flexible functional form that combines a global hybrid meta-nonseparable gradient approximation that depends on density and occupied orbitals with a damped dispersion term that depends on geometry. In the spirit of machine learning, we optimized the energy functional by using a big database and active learning. The results illustrate the power of such an approach. As compared to selected but diverse previous functionals we find excellent performance. The new functional has the best overall performance for the GMTKN55 database (consisting of 55 diverse datasets) with a mean unsigned error (MUE) of 1.45 kcal/mol, and it performs well for both radical systems and nonradical systems in the GMTKN55 database. The CF22D functional gives competitive performance to the recently developed deep-learning functional DM21 from DeepMind (Science 374, 1385–1389 (2021)). It gives excellent performance for the AME418 dataset of Minnesota Database 2019 with an MUE of 2.10 kcal/mol, and it is competitive with ωB97M-V for the MGCDB84 database, especially for noncovalent ‘difficult’ systems and thermochemistry. We combined all the data points used in databases with more transition metal data to create a large, combined database DDB22 (Diverse Database 2022). For DDB22, among the compared functionals, CF22D gives the best overall results for barrier heights, isomerization energies, thermochemistry, and noncovalent interactions and the best results for the transition metal datasets that were used only for testing. Furthermore, CF22D provides the long-range van der Waals tail of potential energy curves for noncovalent interactions, and it gives good performance for vertical excitation energies, dipole moments, and molecular structures. Overall, CF22D is superior to most widely used functionals for a broad range of databases.
CF22D can be recommended for applications involving a broad range of bonding and noncovalent interactions of both main-group compounds and transition-metal compounds, which makes it appropriate for studies of catalysis, functional materials, biochemistry, and environmental chemistry.
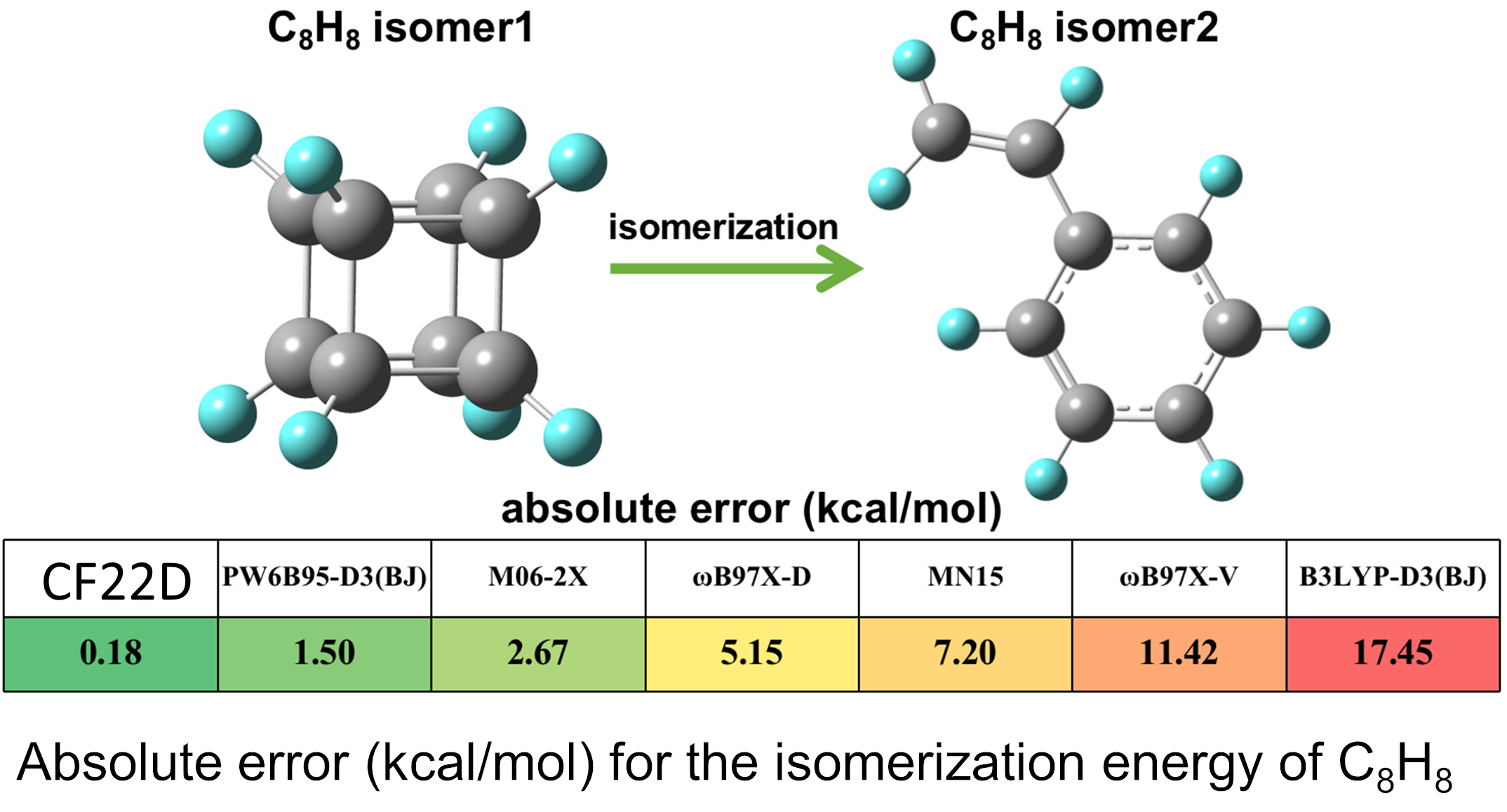
On December 13, Siriluk Kanchankungwankul defended her these entitled “Electronic Structure Theory and Computations: Application of Density Functional Theory to Heterogeneous Catalysis and Density Functional Development.” Siri is moving on to a position at DuPont Silicon Valley Technology & Innovation Center in Sunnyvale, CA.
On December 16, Jiaxin Ning defended her these entitled “Electronic Structures of Lanthanide Compounds and Aromatic Molecules.” Jiaxin is moving on to a position in Research & Development at Bytedance Inc. in Mountain View, CA.
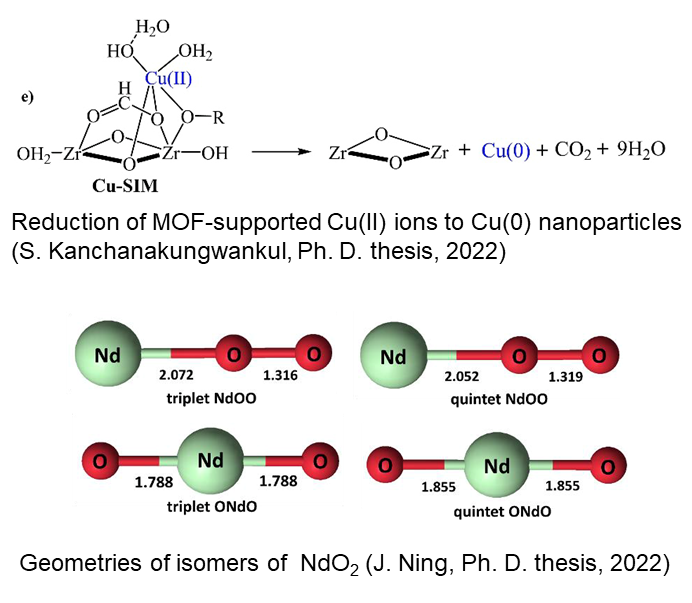
MINNEAPOLIS / ST. PAUL (05/13/2022)—In the first edition of Research.com’s top scientists ranking, seven current Department of Chemistry faculty were included in the disciplines of Chemistry and Materials Science.
Professor Donald Truhlar led the way, with a ranking of #14 in the world and #9 in the United States in Chemistry. He is joined by Professors Lawrence Que, Marc Hillmyer, Timothy Lodge, Jiali Gao, Andreas Stein, and Ilja Siepmann.
According to Research.com, the ranking is based on a meticulous examination of scientists on Google Scholar and Microsoft Academic Graph. For scholars to be included in the list, their h-index must be above 40. Their contributions made within the given discipline, as well as awards and achievements, are also considered. Each profile is then manually verified and cross-correlated against publications in a wide range of credible sources.
Over 35,754 profiles were examined for the discipline of Chemistry, and more than 11,163 were reviewed for Materials Science.
Position in the ranking is based on each scientist’s h-index. The h-index, publications, and citations values displayed in the tables were collected on December 6th, 2021.
Discipline
|
World
|
National
|
Professor
|
H-index
|
Citations
|
Publications
|
---|---|---|---|---|---|---|
Chemistry
|
14
|
9
|
Donald Truhlar
|
172
|
169,433
|
1,149
|
Chemistry
|
143
|
83
|
Lawrence Que
|
124
|
54,129
|
534
|
Materials Science
|
260
|
114
|
Marc Hillmyer
|
111
|
39,988
|
421
|
Chemistry
|
295
|
152
|
Marc Hillmyer
|
110
|
39,127
|
425
|
Materials Science
|
526
|
215
|
Timothy Lodge
|
94
|
34,585
|
441
|
Chemistry
|
717
|
330
|
Timothy Lodge
|
93
|
31,164
|
422
|
Chemistry
|
899
|
397
|
Jiali Gao
|
89
|
44,209
|
263
|
Materials Science
|
1507
|
522
|
Andreas Stein
|
73
|
25,050
|
199
|
Chemistry
|
2207
|
845
|
Ilja Siepmann
|
73
|
19,780
|
217
|
Three department affiliated faculty from the Department of Chemical Engineering and Materials Science (CEMS) were also ranked as top scientists.
Discipline
|
World
|
National
|
Professor
|
H-index
|
Citations
|
Publications
|
---|---|---|---|---|---|---|
Materials Science
|
182
|
84
|
Frank S. Bates
|
120
|
60,767
|
482
|
Chemistry
|
183
|
104
|
Frank S. Bates
|
118
|
59,039
|
430
|
Materials Science
|
802
|
309
|
C. Daniel Frisbie
|
85
|
28,334
|
223
|
Chemistry
|
1585
|
633
|
Matthew Neurock
|
79
|
19,983
|
246
|
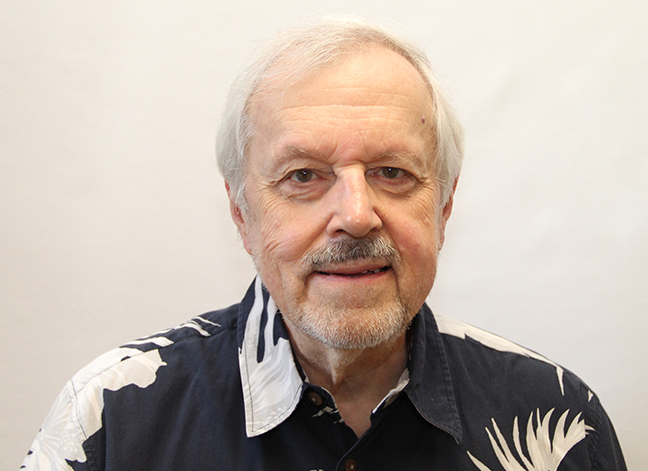
This news article was originally published on the Department of Chemistry News.
Our feature article on diabatic states has now been published:
“Diabatic States of Molecules,” Y. Shu, Z. Varga, S. Kanchanakungwankul, L. Zhang, and D. G. Truhlar, Journal of Physical Chemistry A 126, 992-1018 (2022). doi.org/10.1021/acs.jpca.1c10583
Quantitative simulations of electronically nonadiabatic molecular processes require both accurate dynamics algorithms and accurate electronic structure information. Very often semiclassical nonadiabatic dynamics is performed in a direct way, in which one evaluates the adiabatic potentials, gradients, and couplings from electronic structure at every time step. Direct semiclassical nonadiabatic dynamics is expensive due to the high cost of electronic structure calculations. And hence it is limited to small systems or lower levels of electronic structure methods. In addition, there is an increasing interest in involving nuclear quantum effects. The goals of performing electronically nonadiabatic quantum dynamics with quantitatively accurate electronic structure input and performing electronically nonadiabatic semiclassical dynamics with high levels of theory, long simulation times, and sufficient ensemble averaging have stimulated the development of diabatic representations. Diabatic representations, unlike adiabatic representations, are not unique. But they are very convenient because, in a diabatic representation, the population transfer between electronic states is governed by the diabatic coupling, which – unlike the coupling vector in the adiabatic representation – is a smooth scalar; therefore diabatic potential energy matrices can be fitted to smooth analytic functions. The goal of this article is to review the utility of diabatic representations for dynamics, the characteristics of a diabatic representation, the connections between diabatization methods, and the recent developments of new methods from our group.

On Oct. 9-12, 2021, the Chinese Chemical Society National Conference on Quantum Chemistry was held in Shanghai. Several group alumni who participated are shown in the accompanying picture, which shows, left to right: Xiao He, Xuefei Xu, Yan Zhao, Xin Zhang, and Xin-Ping Wu. The titles of the presentations they made were as follows:
- Xiao He: “Towards Accurate Simulation of Complex Systems”
- Xin-Ping Wu: “Developing QM/MM Methods for Metal-Organic Frameworks”
- Xuefei Xu: “Theoretical and Computational Study of Gas-Phase Chemical Reaction Dynamics”
- Yan Zhao: “Development of Quantum Chemical Methods and their Applications to Energy and Environmental Materials”
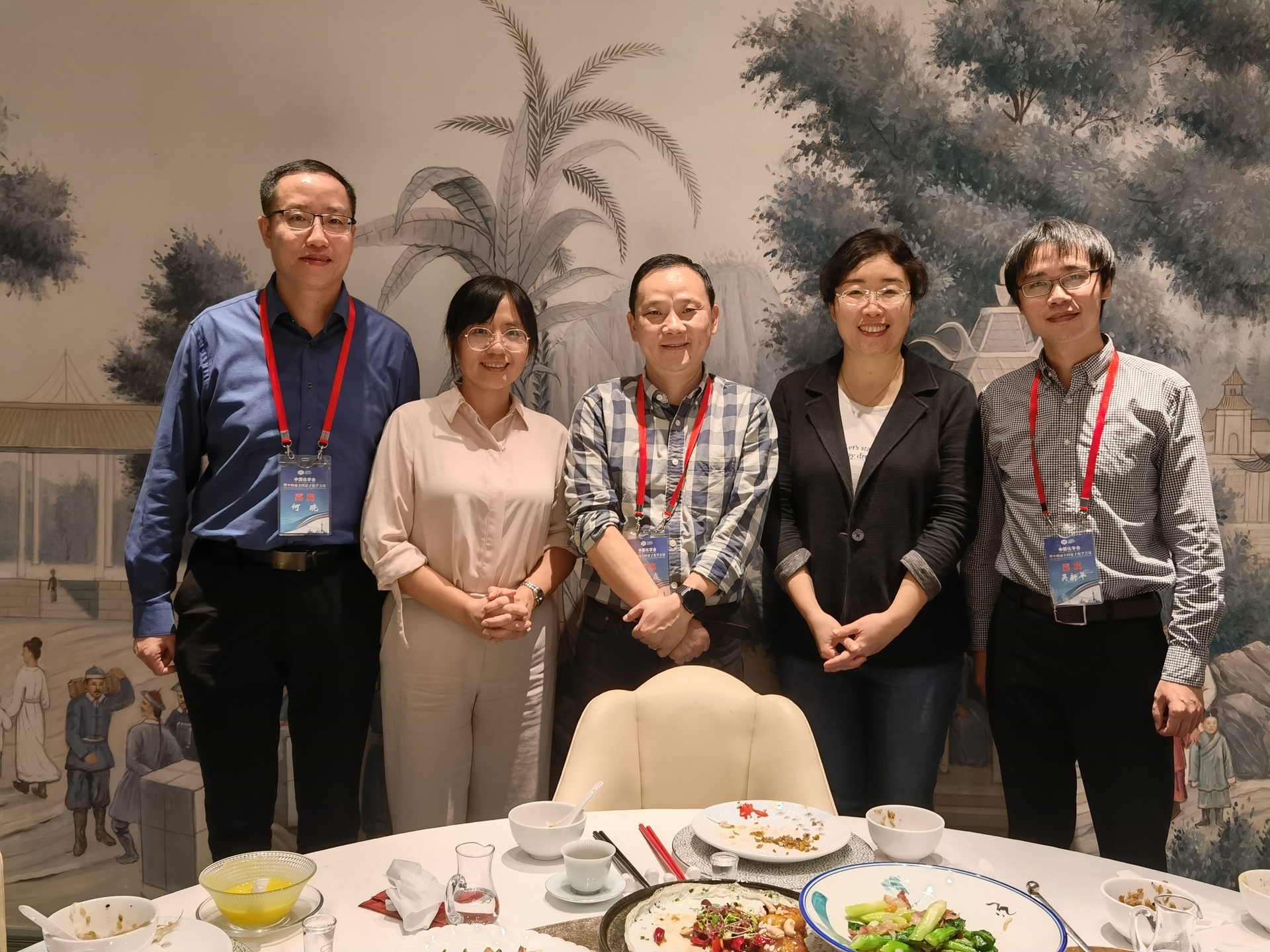